INTRODUCTION
Astronomy has taken a giant step thanks to the development of new
technology in building large optical telescopes, radio telescopes and
sensitive detectors. Cosmic radiation has been observed over a wide band
of the electromagnetic spectrum, from the
gamma rays of ultra short wavelengths
through the visible and infrared radiation to the long radio waves.
Optical astronomy dated back to the epoch of Galileo Galilei, while
gamma astronomy,
infrared astronomy
and radio astronomy have
been developed only in the last century. Multi-wavelength observations
are necessary to understand the nature of astronomical objects. The hot
environment around black holes emits energetic radiation in the form of
gamma and X
rays. Interstellar dust grains heated by stellar radiation emit in the
infrared. Bright nebulae and supernova remnants in our Galaxy are strong
radio sources. With large telescopes and radio telescopes on the ground
together with the instruments launched in space, astronomers can probe
deeply in the universe and make spectacular discoveries.
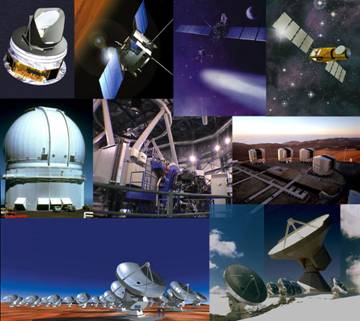
A wide assortment of large telescopes and radio
telescopes installed on the ground and launched in space is now
available to explore the universe (CNRS).
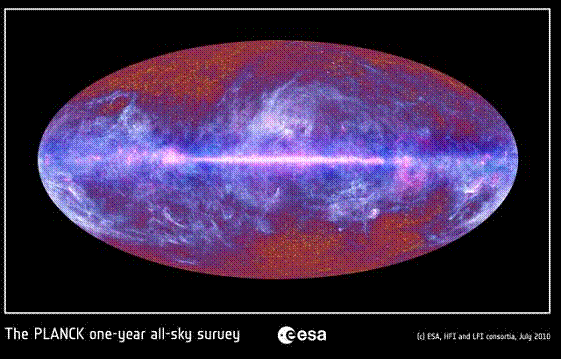
The sky observed in the infrared
by the Planck satellite. Bright thin lane in the middle is due to the
emission of hot dust grains in the plane of our Galaxy. Streamers of
cold dust spread above and below the galactic plane. This dusty
environment is the favourite site of star formation.
Radio
emission of extraterrestrial origin was first detected unexpectedly by
Karl Jansky in 1933, while he was doing the research in the field of
radio communication at Bell Laboratories (USA). Jansky was intrigued to
detect in his antenna a “star noise”, which he interpreted later as
coming from the Milky Way.
A
serendipitous but very important discovery in radio astronomy was the
detection of the Cosmic Microwave Background Radiation. This discovery
provides an experimental proof of the Big Bang theory. Quasars which can
harbour black holes in their centre are the most remote radio emitters
in the universe. Therefore, they can also be used for cosmological
purposes.
The
observation of the 21 cm line emitted by neutral atomic hydrogen
revealed that the diffuse band of light stretching across the night sky,
the Milky Way, is in fact a spiral galaxy as are myriads of others in
the universe.
Galaxies and supernova remnants are the most powerful synchrotron radio
sources. Synchrotron radiation is a non-thermal process
produced by the interaction of relativistic (high energy) electrons
with a magnetic field. The
radio emission from pulsars originates in a rotating core, the remnant
of a supernova explosion. Pulsars appear to be excellent laboratories to
produce gravitational waves. The system of a binary pulsar consisting of
two neutron stars revolving around each other should emit gravitational
waves, according to Einstein’s theory of gravitation. For the first
time, the monitoring of the radio pulses of a binary pulsar led to the
detection of these waves.
The
discovery of interstellar molecules at radio wavelengths also marked an
era in modern astronomy by creating the new branch of astrochemistry.
Some molecular clouds are powerful cosmic masers capable of amplifying
the background radiation by several orders of magnitude.
THE
COSMIC MICROWAVE BACKGROUND
One of
the very important contributions of radio astronomy is the serendipitous
discovery of the Cosmic Microwave Background Radiation at the wavelength
of 7.35 cm by Penzias and Wilson in 1965. This ocean of radiation
spreads over the entire sky and appears to be the remnant of a much
hotter and opaque universe created some 13.7 billion years ago. The
intensity of this thermal radiation is governed by the Planck formula:
I(n)
= (2hn3/c2)
/ {exp (hn/kT)
- 1}-1
n
is the frequency,
c
the velocity of light, h and k are the Planck and
Boltzmann constants.
The spectrum of this radiation fits perfectly the black-body spectrum
at 2.725 Kelvin and peaks at the wavelength ~ 2 millimetres (
n
~ 160 Gigahertz). Subsequent detailed observations by the
satellites COBE and WMAP at millimetre wavelengths with high spatial
resolutions revealed tiny temperature fluctuations ~ 0.0002 degrees
around the average temperature of 2.725 Kelvin, indicating that the
universe was clumpy. Statistical analyses of the temperature
fluctuations obtained at different frequencies together with
cosmological theories gave valuable information on the evolution of the
primordial universe and revealed the existence of a spectacularly large
amount of dark energy and dark matter.

The Microwave Background Radiation observed by the probe
WMAP. The temperature fluctuations are +/- 0.0002 Kelvin around the
average temperature of 2.725 Kelvin (NASA/WMAP Science Team).
THE
21 CM LINE OF ATOMIC HYDROGENThe
energy of an atom and molecule is quantized into a series of discrete
levels. The energy jumps from one level to another according to the
energetic arrangement produced by the change of the electronic states in
the atom or the rotation and vibration of the molecule.
The
most common line emission is the 21 cm line of neutral atomic hydrogen.
This line arises from the transition between two hyperfine levels in the
ground state. The hyperfine transition is due to the change of
the spins of the electron and the proton from the parallel state
to the antiparallel state. Since the energy difference between
the hyperfine levels is small, the radiation is emitted in the radio
range (low energy radiation) at the wavelength of 21cm. For a single
atom, a spontaneous change from the upper state to the lower state is
very rare, since it occurs every 11 million years. Collisions with other
particles can trigger an induced emission and enhance the emission rate.
Since hydrogen is the most abundant constituent of the interstellar gas,
the 21cm emission appears to be the strongest thermal line emission
observed in galaxies. The 21cm line is ubiquitous in the interstellar
medium. It was used to reveal for the first time the spiral structure of
the Milky Way and to detect remote galaxies. The observations of the
Cosmic Microwave Background suggest that most of the matter in the
universe should be in the form of invisible dark matter. The outer part
of the rotation curve of galaxies observed with the 21 cm line of atomic
hydrogen decreases as a function of the distance
r
to the galaxy
centre more slowly than predicted by the Kepler law 1/r1/2.
The anomalous shape of the rotation curve of galaxies indicates that
there should be a halo of invisible matter around the galaxies and
provide additional clues for the existence of dark matter.
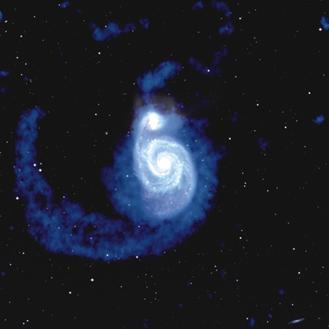
Composite
image of Whirlpool galaxy (Messier 51). Radio emission from neutral
hydrogen (faint feature
at large distance) extends well beyond the optical galaxy (bright
spiral arms).
(Courtesy
NRAO/AUI; Juan M. Uson).
QUASARS AND SUPERLUMINAL MOTION
Quasars
are extremely compact and intrinsically very bright. They are thousands
of times more luminous than the brightest galaxies, yet they have the
appearance of very faint stars because of their large distance.
Therefore, it is difficult to find them in the optical sky, since they
are easily confused with billions of other stars in the Milky Way. Some
quasars are strong synchrotron radio sources and appear extremely bright
in the radio sky. This is the reason why the first optical quasars were
identified with the help of their radio counterparts.
Sporadic explosions in quasars eject clouds of relativistic electrons
emitting synchrotron radiation. The intensity of the quasar is highly
variable. There should be a time lag for the radiation to reach the
earth from the back side and from the front size of the quasar. From the
measured variability time scale
t, one can determine the “light
travel distance”
ct, assuming that the ejection occurs at the
velocity of light
c. Thus, this value corresponds to the maximum
size of the quasar, which turns out to be only about 10 light-hours, the
size of the solar system. It is extremely small compared to the size of
a galaxy which is about a hundred thousand light-years. Synchrotron
radio emission can be used to investigate the core of the quasars, which
is the central engine producing a tremendous amount of energy.
The
electron clouds ejected from the quasars move at relativistic velocities
(close to the velocity of light). The apparent cloud velocities
projected onto the plane of the sky can be much larger than the velocity
of light (superluminal velocities), though their true velocities are
subluminal. The paradox of superluminal motion can be explained by the
theory of special relativity.
THE
COSMIC MIRAGE
General
relativity predicts that the radiation from a remote galaxy or quasar
can be deflected by the gravitational field of an intervening massive
object lying by chance along the line of sight. The intervening object
can be a galaxy or a cluster of galaxies. The gravitational lensing
mechanism is similar to the focusing effect of an optical lens.
The
discovery of a twin optical quasar which is also a double radio source
raised the question about its origin. The two components are separated
from each other by only a few arcseconds. Furthermore, the fact that
they have the same redshift (the same distance) implies that they are
very close in space. They are actually the images of a very faint single
quasar at a distance of ~ 10 billion light-years. The remote quasar
happens to be almost aligned with a foreground galaxy located at about
half way between us and the quasar.
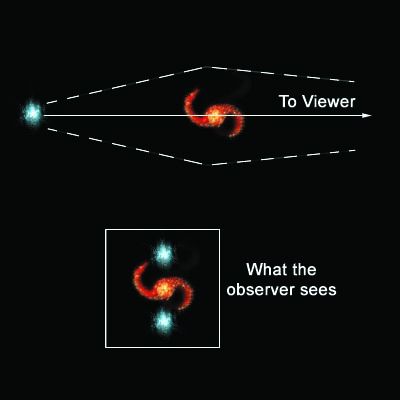
Upper:
the gravitation field of a foreground galaxy (red spiral) bends the
light of the background quasar (blue dot).
Lower: this
phenomenon gives rise to a double quasar image (blue
dots).
Gravitational lensing not
only amplifies the background object, but also distorts its image. It
creates a cosmic mirage depending on the more or less complex
distribution of the galaxies in the cluster present in the foreground.
If the foreground and the background objects are single and perfectly
aligned along the line of sight, the lensing effect results in a ring,
the “Einstein ring”, surrounding the position of the objects in the sky.
The radius of the ring is r = (4GMd/c2)1/2,
where G is the gravitational constant, M the mass of the
deflector and d a function of the distance of the deflector to
the background source and to the observer.
Upper:
A distant galaxy 1938+ 666 appears in the infrared as a perfect Einstein
ring around the lensing galaxy (bright spot in the centre of the ring).
Lower:
The radio image of this galaxy is an arc instead of a complete ring,
since the radio counterpart is not perfectly aligned with the lensing
galaxy along the line of sight.
(Images: Hubble
telescope and MERLIN).
The lensing effect can be
used to investigate intergalactic clouds of dark matter and remote
galaxies in formation which would remain otherwise undetectable.
INTERSTELLAR MOLECULES AND ASTROCHEMISTRY
Molecules emit spectral lines when they rotate and vibrate in space. The
excitation of low-lying rotational and vibrational energy levels leads
to the emission of radio and IR lines. Hydrogen in the
interstellar dense clouds is almost entirely in molecular form.
Therefore, the 21 cm line of atomic hydrogen cannot be used as a tracer
of molecular clouds. The discovery of interstellar molecules has been
helpful to probe this very important component of the interstellar gas.
The first interstellar diatomic molecules CH, CN and CH+ were
detected in 1937 – 1941 through the ultraviolet absorption lines. The
detection of diatomic OH molecules in space at radio wavelengths was
made in 1963. These 18 cm OH transitions arise between two hyperfine
levels of a doublet in the ground energy state. Radio lines are easier
to excite than optical lines because they arise from low-lying molecular
levels. The improvement of radio astronomical techniques resulted later
in the detection of polyatomic molecules, such as NH3, H2O,
… So far, about 150 molecular species, mostly organic
compounds, have been detected in space at millimetre wavelengths. The
heaviest molecule ever detected is HC11N belonging to the
long-chain carbon molecules, the cyanopolyynes HC2n+1N.
In an
attempt through experiments in the laboratory to understand the
mechanisms of formation of cyanopolyynes in space, chemists discovered
fullerene, a very stable molecule made of carbon atoms distributed on
the surface of a cage consisting of polygons with 60 vertices and 32
faces. A carbon atom is placed at each vertex of this structure. The
discovery of fullerene has an important impact on nanotechnology.
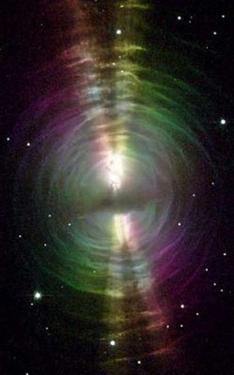
The Egg Nebula: a star is hidden in the central dusty
disk (dark lane in the middle) and diffuses its light through a hole
(NASA).
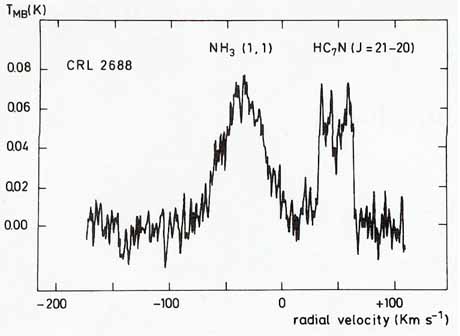
Spectral lines of ammonia and cyanopolyyne HC7N
detected in the Egg Nebula (Nguyen Quang
Rieu, David Graham, Valentin Bujarrabal; Astron. Asrophys. Vol. 138,
1984).
Since acids and amines have been detected separately in the interstellar
medium, it is tempting to search for amino acids. Glycine (NH2CH2COOH),
the simplest amino acid may be the best candidate.
Searches for interstellar glycine began as soon as the frequencies of
radio lines were measured in the laboratory. Since three decades, many
attempts at detecting glycine, including our attempt towards the centre
of our Galaxy and the Orion Nebula, turned out to be unsuccessful. The
glycine lines are indeed very weak and their spectrum can be
contaminated by other faint molecular lines. Scientists at NASA
announced recently that they detected glycine in a sample of dust
gathered in the tail of a comet. Radio astronomers detected interstellar
amino acetonitrile (NH2CH2CN) which may be a
precursor of glycine.
Searches for prebiotic molecules are very important in astrochemistry,
since they are the building blocks of living organisms. The detection of
these molecules would have a great impact not only on interstellar
chemistry, but also on the origin of life and the possibility for
extraterrestrial life to exist. Life can be present on planets with
appropriate atmospheric conditions. Hundreds of extraterrestrial planets
have been detected and intense searches are under way to find rocky
planets similar to the earth.
MASER ACTION IN SPACE
Radio
astronomical observations have led to the discovery of unexpected and
spectacular phenomena in physics. One of these is the ability for the
dense molecular clouds harbouring stars in formation or the envelope of
old dying stars to greatly amplify the background radiation. This maser
action is a well-known phenomenon in the laboratory. Masers are built to
amplify weak radio signals received on radio telescopes. In space, the
clouds containing H2O, OH and SiO molecules are the most
powerful masers. Methanol (CH3OH) and silicon sulphide (SiS)
exhibit weaker maser emission.
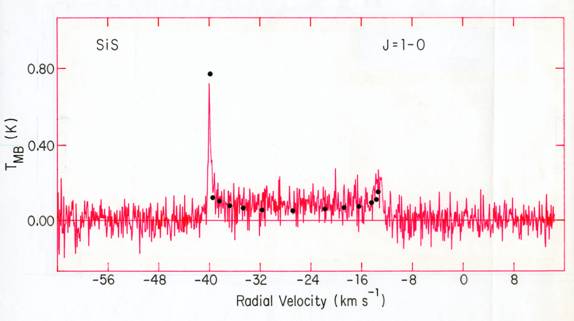
The SiS maser from the envelope of the star IRC+10216
was detected as a spike (on the left in the spectrum) whose intensity
varies in time. Black dots represent a synthetic spectrum calculated
with a theoretical model to fit the observed spectrum in order to
determine the physical parameters inside the stellar envelope. (Nguyen
Quang Rieu, Valentin Bujarrabal, Hans Olofsson, Lars Johansson and Barry
Turner; Astrophys. Journal., Vol 286, 1984).
Interstellar clouds are in general in “local thermodynamic
equilibrium” (LTE) state, in which the population n of
molecules is governed, according to their energy E, by the
Boltzmann law: n ~ exp(-En/kT). A maser effect is
produced when the population of molecules is not in the LTE state. This
situation occurs when the molecules are pumped to higher energy levels
by collisions with other particles or by the infrared photons from stars
and warm interstellar dust grains. This population inversion results in
an excess of molecules in high energy states as compared to the LTE
population distribution. The maser emission is then induced by photons
inside the cloud or by an external radiation. The investigation of the
interstellar maser emission requires the resolution of the statistical
equilibrium equations, which control the level populations, coupled with
the radiative transfer equations, which describe the intensity of the
radiation during its propagation through the cloud.
ASTRONOMY IN VIETNAM
In tropical countries like Vietnam,
the humid and hot climate is not favourable to optical observations. Cosmic
radio waves at centimetre and longer wavelengths propagate almost freely through
the atmosphere even saturated with water vapour. Therefore in such an
environment, radio astronomy would be appropriate to the observation of the sky.
This was the choice of Indian astrophysicists who built a Giant Metrewave Radio
Telescope (GMRT) near the city of Pune. This interferometer consists of thirty
45-m antennae and operates from 150 MHz (l
= 2 m) to 1500 MHz (20 cm).
It can be used to detect remote embryonic galaxies formed at the very early
stage. Their 21 cm line emission is expected to be redshifted to metre
wavelengths due the expansion of the universe.
After three decades of wartime,
Vietnam was faced with many important problems of economy. Nowadays it seems
eager to develop both applied and fundamental sciences. Space science is one of
the fields having priority. At the beginning it is not necessary to build large
radio telescopes. Astronomers of any country are allowed to use the instruments
built abroad if they have reasonable scientific proposals. In principle, they
can observe remotely from their laboratory and reduce their data without being
present at the telescope site. Model calculations can also be done with
computers to interpret the observations.
Astrophysics involves
several fields from physics and chemistry to mathematics. A concrete measure to
take is to form young astrophysicists inside Vietnam or abroad. A radio
telescope of modest size should be useful for teaching purposes. So far, a few
vietnamese astrophysicists were formed at the Paris Observatory and came back
to the country. They form a core of specialists who should be encouraged to do
research and to teach in the field of astrophysics.
PROSPECTS
Important discoveries in astrophysics have been made. The spatial
resolution of a telescope (the smallest angular separation between two
points in the sky that can be distinguished by the telescope) is ~
l/D,
where l
is the operating wavelength and D is the size of the
instrument. Since radio wavelengths are at least a thousand times larger
than optical wavelengths, radio astronomers must build giant single
antennae of hundreds of metres and interferometers extending over tens
to thousands of kilometres, in order to get a spatial resolution
comparable to optical telescopes. State-of-the-art receivers built with
high electronic technology have been installed at their focus.
The ALMA (Atacama Large Millimetre
and Submillimetre Array) is an interferometer being built and installed on a
desert plateau in Chile at the altitude of 5000 metres. It consists of about
sixty 12-m antennae which can be spread over distances from 150 m to 16 km.
ALMA will be the best ground-based instrument ever built in radio astronomy.
With its spatial resolution ten times better than that of the Hubble Space
Telescope and its large collecting area, the ALMA interferometer will be used to
investigate distant objects at the edge of the observable universe.
The space telescopes Planck and
Herschel launched in 2009 and operating in the far infrared and submillimetre
bands will be devoted to a detailed observation of the Microwave Background
Radiation and stars and galaxies still in formation. These data combined with
those obtained with large optical telescopes are expected to reveal further
important secrets of the primordial universe and the cold world of nascent stars
and galaxies.
Nguyen Quang Rieu
Article
published by the author in the “Proceedings of the
Conference on Nuclear Physics, High Energy Physics and
Astrophysics”
(Hanoi-Vietnam November 2010).